13. Implications and Applications¶
Week 13 | This week we created our project pitches and presented them to the Fabricademy team for review.
• • •
Introduction¶
Using digital fabrication technology and bio-fabrication techniques to design a 3d bio-printed coral reef structure for use in conservation, which is formed from the binding between a living microorganism and natural pollution filtering materials.
Key Words: Bioremediation | 3D Bioprinting | Biocalcification | Regenerative BioDesign | Conservation | Living Structures
• • •
Tools¶
Fabricademy
-
State of the Art
-
Digital Bodies
-
Computational Design
-
BioFabricating Materials
Institute of Advanced Architecture Catalonia (IAAC) & FabLab BCN
-
3D BioPrinters
-
Robotic Arms
-
BioLab
• • •
WHAT¶
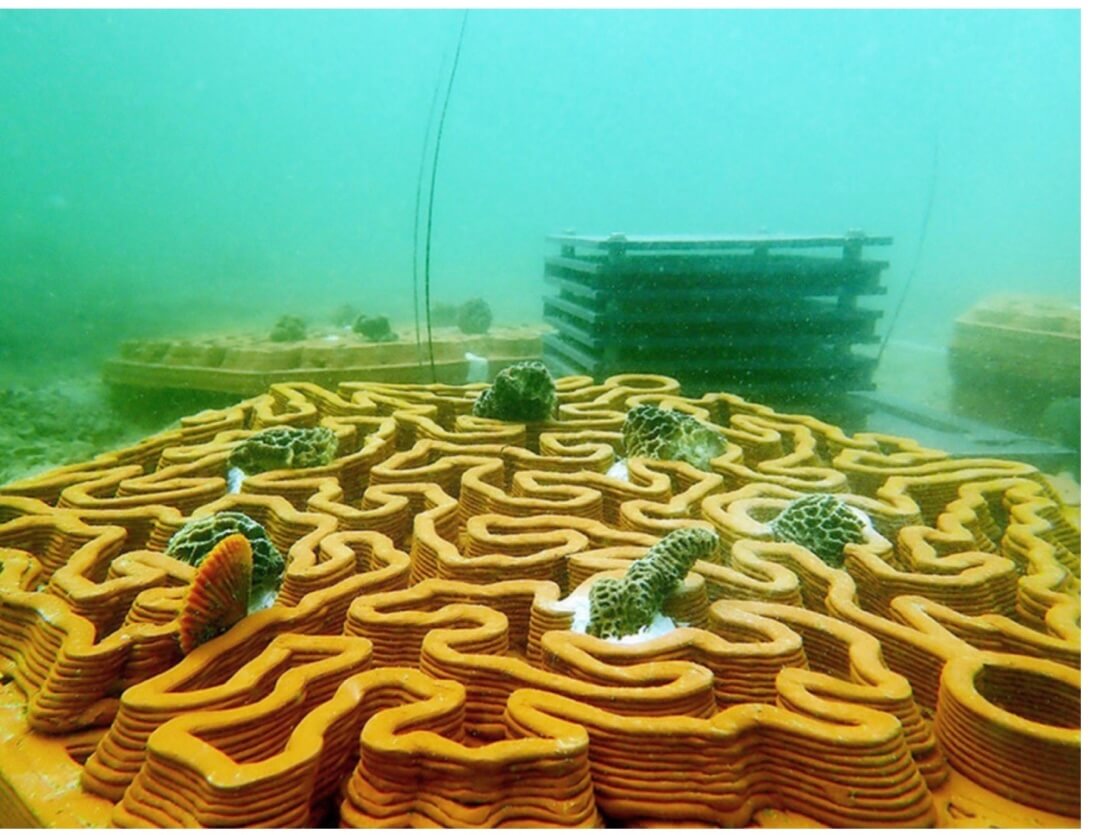
Photo by 3DPrinting on "3D Printed Clay Tile Coral Reef"
I am proposing a 3D bio-printed coral reef structure using living organisms and biomaterials to enhance the reef structure, enabling processes such as biomineralization and bioremediation to occur, pulling pollution from the marine environment and solidifying the reef skeleton. This design provides a more hospitable living environment for marine life and allows for a self-healing living structure which closely imitates coral reefs. The hope is to cultivate marine fungi or cyanobacteria that are adapted to survive in the harsh and changing conditions to create a stronger reef scaffold.
Bioremediation: can either stimulate natural microbes to consume contaminants as their energy and food source, or in the case of materials such as clay, filters pollutants such as heavy metals from water sources.
Biomineralization: occurs when the calcium dissolved in seawater is used to produce solid crystallised marine structures such as skeletons and exoskeletons, fundamental in building the foundation of a coral reef. Cyanobacteria are a great example of a microorganism which precipitate calcium carbonate minerals
• • •
WHY¶
The rapid decline of coral reefs has increased the necessity of exploring interdisciplinary methods for reef restoration. There is an urgency to invest in technology that can help reach ecosystem-scale [1].
Coral Reefs are the most vulnerable ecosystem to climate change, seeing a decline of 50% since the 1950s [1],[5]. The reefs support around 25% of marine life [2]; provide food, coastal protection, and revenue for millions of people [3]; and are a sink for approximately 29% of all CO2 absorbed by the ocean [4]. Projections estimate up to 90% of coral ecosystems will be severely degraded by 2050 [1].
The problems facing current restoration techniques include:
-
a difficulty replicating the 3D complexity of coral habitats
-
difficulty scaling them to larger areas
-
pollution leaching into the environment from toxic objects such as sunken ships or concrete
-
a lack of sustainable restoration methods
• • •
RESEARCH¶
Academic¶
Artificial Reefs: What works and what doesn’t
Doesn’t work: trash and toxic materials; small unsecured structures
Does work: wrecks and steel structures; concrete structures; modular units; mineral accretion devices
Reef Revolution: How implementation of 3D printing can promote sustainable coral restoration
Example of successful 3D printed reefs:
- Archireef - Hong Kong, 95% coral survival rate.
Complexities of design: reefs need to be designed for the particular location, environment and organisms; selection of durable and strong materials e.g clay
Fish were more abundant in corals with the highest levels of complexity. Findings suggest that 3D-printed corals can serve as a complementary tool to improve the ecosystem function of degraded coral reefs.
Organisations¶
Current innovative methods for reef restoration include propagating coral polyps on frames and 3D printing; while traditional methods sunk objects such as ships or concrete blocks.
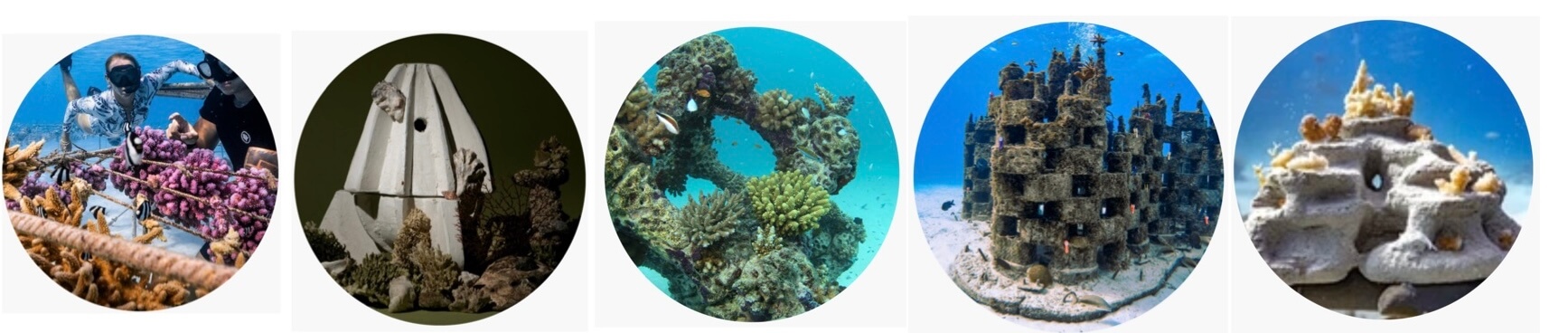
Photo by Coral Gardeners | Photo by Reefscapers | Photo by Reef Design Lab | Photo by Reefscapers | Photo by RRReefs | Photo by Coastruction
Coral Gardeners: Grow coral fragments on rope to revive ecosystems and providing live coral growth tracking information.
Reef Cycle: Use plant enzymes to mimic shell formation and create bio-cement. Resulting in marine-safe material that can be produced in situ without heat, energy, or resource depletion.
Reef Design Lab: 3D printed reef structures made from eco-concrete, oyster shells or other recyled materials.
RRReefs: 3d printing customisable clay reef structures, and empowering communities through science, art and education.
Coastruction: 3d printing using natural materials aiming for the lowest CO2 footprint possible. Ideally, local materials such as beach sand or recycled concrete.
• • •
WHERE¶
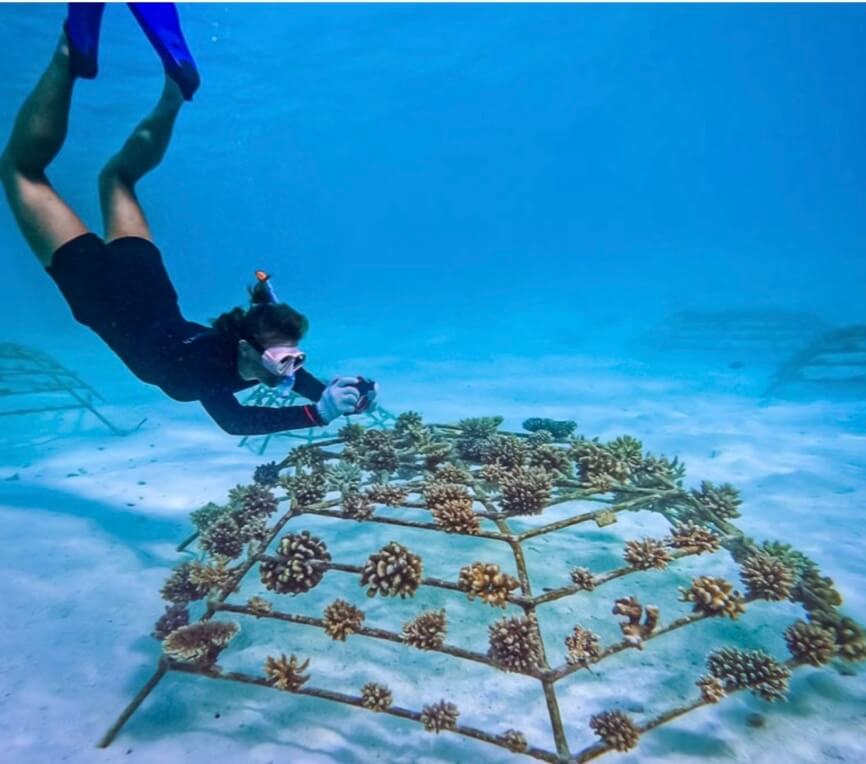
Photo by Reefscapers
Artificial reefs are very location and ecosystem dependent. When designing a reef these factors should be considered:
-
local marine ecosystems and biodiversity
-
water depth, light levels and temperature
-
hydrodynamics (waves, current and sedimentation)
-
seafloor characteristics
-
human and environmental impact
I will use open access resources of downloadable 3D photogrammetry models of coral reefs to identify which part of the world I will study.
Some resources I have found include:
• • •
HOW¶
Relevance/Need¶
Current concrete based methods of reef restoration are still polluting the marine environment and the concrete industry is one of the largest producers of CO2 [5],[6]. Designing a reef made from locally sourced, low carbon footprint biomaterials minimises the release of microplastics or pollutants into the water.
Artificial reefs may also not exist symbiotically with the marine life [5] however, creating a living structure with bioremediation and biomineralisation properties will allow for a more hospital marine environment.
Molded reef structures have less complexity and don’t mimic the natural environment well, yet utilising 3D printing techniques allows for more complex customisable geometries based on data from global reefs. 3D printing also offers tools to digitise 3D images into biomimetic structure[7].
Process¶
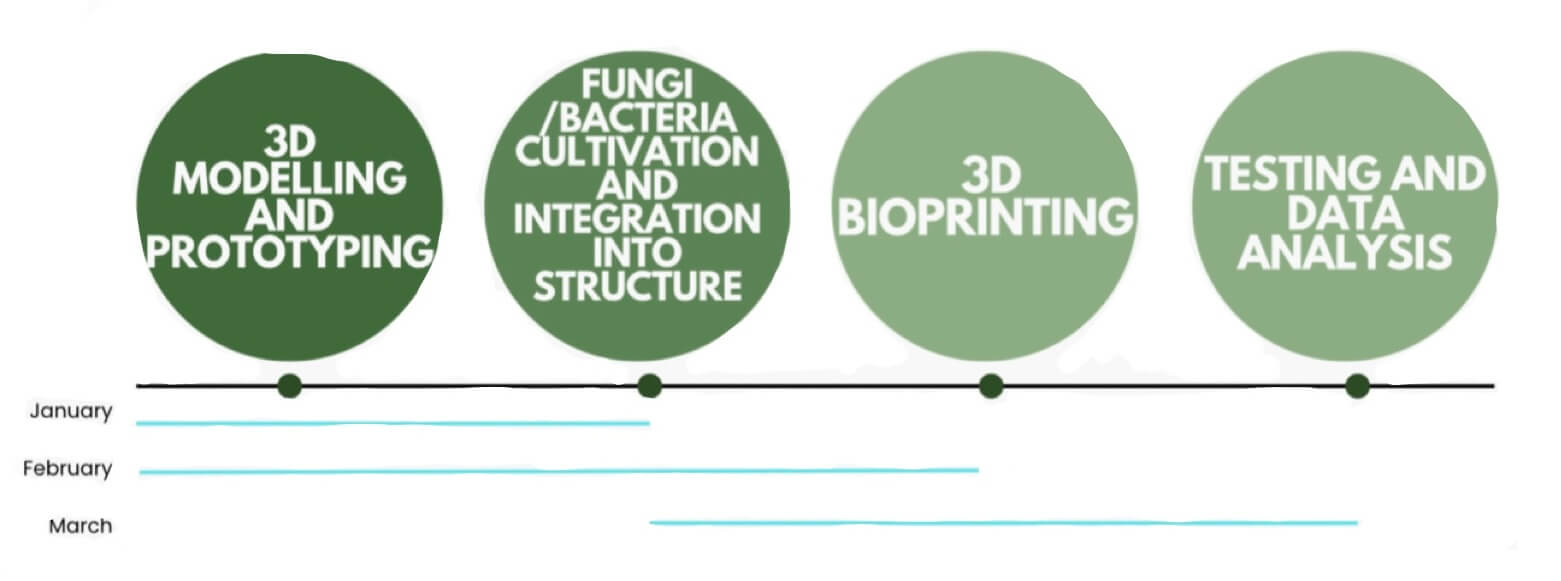
3D MODELLING AND PROTOTYPING (Jan - Feb)
The first stage will be consist of finding a suitable 3D scanned reef model (which has been created via photogrammetry) to either use or base my designs off of. It would be most practical in the time frame to find a 3D model which I can use and adapt for 3D bioprinting. This model would also be much more accurate with geometries that are well integrated into the specific marine environment. I would prototype these models with the base biomaterial such as clay and measure the bioremediation properties.
FUNGI/BACTERIA CULTIVATION AND INTEGRATION INTO THE STRUCTURE (Jan - Feb)
Following research articles and mentoring I will cultivate the living organism and under test conditions such as salinity levels, I will measure how it could be affected by seawater. The living organism would then need to be integrated into the biomaterial before 3D bioprinting and tested again to monitor the biomineralisation properties.
3D BIOPRINTING (Feb - March)
Using the 3D models and composite material consisting of a living organism and biomaterial, the structure will be 3D bio printed and left to complete the process of biomineralisation. I am suspecting that the structure will need to be baked once the calcium carbonate skeleton has been formed, if the living organism does not survive well under seawater conditions.
TESTING AND DATA ANALYSIS (Feb - March Onwards)
Bioremediation properties such as CO2 or heavy metal uptake will be analysed and coral fragment/polyp integration and growth success would be tested and recorded . If the structure were to be deployed to the affected environment, regular data collection would be taken on coral growth, abundance of marine life, resilience of the structure and integration in the ecosystem. A study on how the organisms interact with the material and how they settle on it would also be taken.
• • •
MATERIALS¶
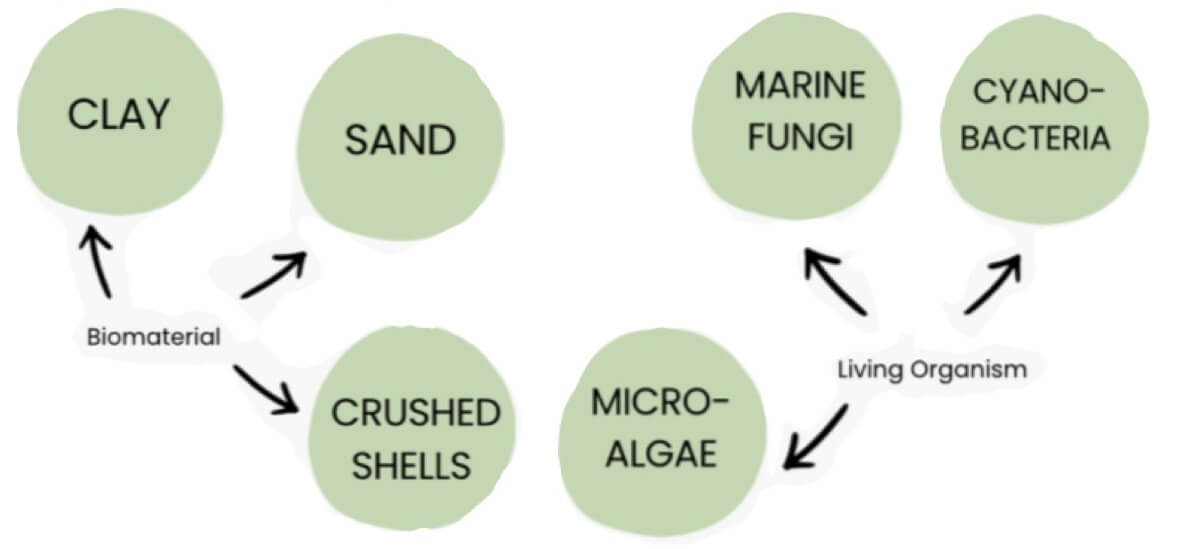
Taking inspiration from design innovations such as self-healing biobricks [8] , 3D printed architecture with living materials [9], and bio-cement [10] I will choose a biomaterial and living organism to build a resilient structure which produces calcium carbonate and filters pollutants from the water.
Other bio-bricks formed from microbial carbonate precipitation such as when urine, sand and bacteria are mixed together, also pose as a potential exploration for this application. The loose sand is colonised with bacteria that produce urease. The urease breaks down the urea in urine while producing calcium carbonate through a complex chemical reaction. This cements the sand into any shape [11].
The living material will either be chosen based on its ability to survive in aquatic environments or its ability to produce calcium carbonate (i.e it will be baked before being submerged)
Reinforcement with steel may be necessary depending on the location.
Biomaterial¶
Clay
Adding clay minerals to marine oil pollution is suggested as an effective and economically interesting way of enhancing the bacterial digestion of contaminating spills. The sediment with the highest surface area and cation exchange capacities induced the highest degree of oil-breakdown [12].
Sand
Bioremediation of contaminated soil with hydrocarbons (7%) was investigated, the soils were inoculated with petroleum degrading microorganisms. Bioremediation was most successful in sandy soil with 70% removal in 270 days. Bioremediation was not successful in clay unless frequent mixing was applied [13]. Using coral sand would be the most efficient and local resource to collect.
Shells
Studies showed that minerals in the crystalline forms of calcite and aragonite have the ability to adsorb metal ions [14].
An alternative and more sustainable method to obtain calcium carbonate is from biogenic waste shells such as poultry eggshells and seashells.
Living Organism¶
Marine Fungi
Marine fungi have unique adaptations to salinity and intense pressures found in the deep ocean. There is potential for marine fungi to help combat our oil spills and microplastics crisis. While marine fungi can survive in seawater, it seems that they do not necessarily prefer it, since there is a cost to maintaining these adaptations. This may provide a chance for the fungi to be cultivated in a dry environment and then deployed into saltwater [15].
Certain marine fungi can perform or contribute to bio-calcification. While bio-calcification is most commonly associated with corals, mollusks, and some algae, fungi may play supportive or indirect roles in this process in marine environments:
CyanoBacteria
Cyanobacteria tend to prefer growth in a slightly alkaline environment transferring cyanobacteria to a higher pH environment is not expected to impact cell viability or growth significantly. This adaptation enables high-density cultures to be grown and then transferred to a more alkaline environment for continuous microbially induced carbonate precipitation. Microbially induced calcium carbonate precipitation has been demonstrated to improve the strength and durability of cementitious materials, concrete self-repair, and crack sealing [16].
Micro Algae
Prominent examples for bioremediation in aquatic environments are the use of microalgae that accumulate heavy metals https://www.frontiersin.org/journals/marine-science/articles/10.3389/fmars.2023.1070905/full
• • •
CONCLUSION¶
“3D bio-printed coral reef structure enhanced with living organisms and sustainable biomaterials with bioremediation and biomineralization properties for reef restoration and increased biodiversity”
⟡ There is little to none of research into 3D bio-printing a living coral structure using e.g clay and cyanobacteria which would promote biocalcification and bioremediation within the skeleton.
⟡ 3D bio-printing will allow for more complex geometries, easier customisation with 3D modelling and photogrammetry, and higher surface area for larvae attachment
• • •
References¶
[1] Emerging 3D technologies for future reformation of coral reefs
[2] World Corals Are Bleaching
[5] The Eco Friendly Alternatives to Ocean Concrete
[6] Biocement Fabrication and Design Application for a Sustainable Urban Area
[8] Self Healing Bricks Eat CO2
[10] BioCement Fabrication and Design Application
[11] Bio-Brick made from sand, urine and bacteria
[12] Clay mineral enhanced bioremediation of marine oil pollution
[13] The effect of soil type on the bioremediation of petroleum, contaminated soils
[14] Seashell Adsorption behaviours in cement-based materials
[15] Superpowers of marine fungi
Fabrication Files¶
-
File: Presentation .pdf ↩